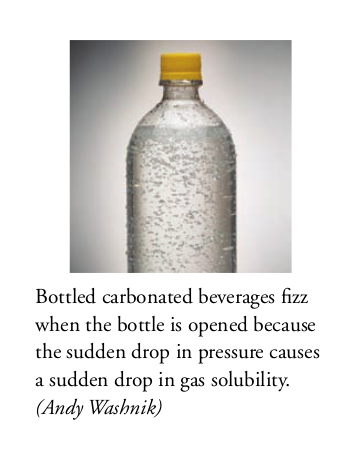
- Henry’s Law does not apply to the chemical reactions and chemical reaction products which begin with and are subsequent to the ionization of CO2 gas with water ions. Henry’s Law does not apply to H2CO3, CO3-², HCO3 and the hundreds of other carbonate reactions that occur in seawater and biosphere which occur subsequent to the ionization of CO2 gas in water. The Henry’s Law constant is a physical partition ratio of a specific gas (i.e., the solute gas) dissolved in a specific liquid (i.e., the liquid solvent) in continuous contact with the same solute gas immediately above the same liquid surface; Henry’s Law defines a physical phase-state partition, like a melting point or boiling point. Henry’s Law does not define chemical reactions, but defines the ratio of a change of phase of a gas in a gas matrix versus the same gas in a liquid matrix.
- Henry Law applies to all natural gases and liquids. For our interest here, Henry’s Law applies to the approximately 1% or less of CO2 gas which diffused into and is absorbed into water, and that ~1% is not ionized, is electrically neutral, uncharged. It is “approximately” 1% because the exact percentage depends on the temperature of the water. Temporarily local conditions in the water including alkalinity, pH, salinity, temporarily change CO2 gas concentration in the local conditions by changing the equilibrium of the several carbonate chemical reactions, similar to adding salt to boiling water to raise the temperature of the water.
- Henry’s Law only applies to solute gases which are in minor concentrations relative to the gas matrix and relative to the liquid matrix. For avoidance of doubt, 400 ppm or 5000 ppm CO2 gas in air or water are minor concentrations. A solute is defined as a minor component dissolved in a liquid.
- The Henry’s Law equilibrium is always a dynamic equilibrium. It is never a static equilibrium as an equilibrium is commonly considered because the temperature of natural waters such as the ocean, or a bubble, or a raindrop, are never static, thus the Henry’s law ratio is never static. There is no average temperature of earth’s surface, nor ocean surface, nor atmosphere, nor column of ocean water, these are calculations not measurable within the uncertainty required by science, thus there is no average Henry’s Law constant for a specific combination of liquid and gas. There is no global Henry’s Law constant for CO2 and ocean. The Henry’s Law constant in polar latitudes is different from tropical latitudes due to different sea surface temperatures.
- Le Chatelier’s Principle is the basis for all processes of absorption and emission of gases into and out of all liquids. A liquid-gas system which is not in equilibrium tends to approach equilibrium. Thus, if the liquid is not saturated with a gas under the existing conditions, then the liquid tends to absorb a gas at a higher rate than it emits a gas. Thus the Henry’s Law coefficient or constant describes the limiting condition, a condition which is always being approached but never achieved because CO2 gas reacts (hydrates) with water ions and those ions subsequently react with other ions and molecules in the water matrix. The result is CO2 is being continuously but converted to various forms of carbonate, dominantly one of the forms of calcium carbonate since calcium ions are about 4 times as abundant as the bicarbonate, carbonate and carbonic acid combined in the well mix layers of ocean, and then slowly precipitated as solid incorporated in stone or structure of coral, sea shells, or sea creatures. With about 4 times more calcium ions as carbonate ions, ocean is only saturated with CO2 gas in temporary and local conditions and when those conditions subside to absorption of CO2 gas will begin again to approach equilibrium. Unless some catastrophic geological or astronomic event occurs that overcomes the multiple chemical buffering systems in ocean to dramatically alter pH, alkalinity, or salinity, then it ocean will be an infinite and perpetual sink for absorbing all CO2 up to the limit of the Henry’s Law ratio.
- Henry’s Law is an intensive property of matter, like a boiling point, a melting point or a specific heat. The defining characteristic of an intensive property of matter is: the amount of matter does not change the constant. Henry’s Law is an Arrhenius Constant, which means the constant varies with temperature. The amount of CO2 added to the atmosphere by any source natural or human, and the amount of CO2 absorbed into any sink natural or human, does not change the Henry Law constant at a given temperature. Henry’s constants are determined by the specific gas solute, the specific liquid solvent and the specific temperature at the gas/liquid interface. Henry’s Law is valid for all known solute gas and solvent liquid combinations, not only CO2 gas and seawater.
- Henry’s Law constants are ratios of the molar mass of the unreacted solute gas which is diffused into the liquid divided by the molar mass of the unreacted solute gas in the air immediately above the liquid/gas interface surface. Henry’s Law constants are intensive properties of matter because the diffusion rate of any solute gas into any liquid solvent is inversely proportional to the square root of the molecular weight of the solute gas, and the molecular weight of a gas is a constant; this is Graham’s Law. Solubilizing a gas follows diffusion of the gas into the surface.
- Henry’s Law constants are a function of the temperature of the interface surface between the gas and the liquid, wherever that surface exists. The absorption rate (or solubility) of all gases in all liquids is inversely proportional to the temperature of the liquid surface. Emission of the gas from the liquid surface is directly proportional to the temperature of the liquid surface. The surface interface may be as vast as the surface of the ocean, i.e. 71% of earth’s surface, or as small as the surface of a small bubble of gas released deep in the ocean, or the surface of a raindrop condensing in a cloud. All of this paragraph applies to all atmospheric gases, not only CO2.
- Unfortunately, many textbooks and science papers merge aqueous CO2 gas with carbonic acid and this causes misunderstanding and error regarding Henry’s Law. Henry’s Law does not apply to carbonic acid, but does apply to aqueous CO2 gas. In these unfortunate documents, the two combined chemical entities are usually given a hypothetical nomenclature like CO2* or similar, an entity which does not exist. This is done for hypothetical and theoretical considerations in a supposed static equilibrium which also does not exist; these hypotheticals result in confusion and error regarding Henry’s Law. These hypothetical expressions are used because the CO2 hydration reaction is almost instant, and the reverse reaction is also nearly instant. The forward and reverse reactions occur continuously and simultaneously due to continuously variable temperature changes in the surface water thin layer, changes in alkalinity, pH, salinity, changes partial pressure of the CO2 gas in the water surface thin layer, as well as changes in the gas thin layer immediately above the water surface.
CO2 gas in air (and all other gases in air) are continuously and simultaneously colliding with the surfaces such as ocean at continuously varying collision rates due to variations in air temperature/pressure and variations in CO2 partial pressure. Similarly, CO2 effusion from ocean surface occurs continuously and simultaneously with diffusion into the surface; but the rate of emission and the rate of absorption are not parallel due to the wide difference in density between the liquid matrix and the air matrix and the intermolecular and interionic forces in the liquid thin layer which are negligible or do not exist in the air thin layer matrix above the liquid surface. Thus, effusion into air is faster than diffusion into water. (Description of the chemistry and physics of the water thin layer and air thin layer are interesting and important, involving virial forces between the molecules and ions, van der Wals force, surface tension, but these are beyond the topic here.)
The rapid and reversible CO2 hydration reaction means the products, reactants and activation energies of the CO2 hydration reaction are difficult or impossible to measure with sufficient accuracy or precision. In practice, the molar mass concentration of the CO2 gas in the head space above the liquid surface is quantified by gas chromatography or gas chromatography mass spectrometry and then the molar mass of the CO2 gas in the liquid are calculated by Henry’s Law. Accurate and precise sampling of the reactant CO2 gas and the product carbonic acid in the hydration reaction is extremely difficult if not impossible. For example, Keeling et al and many others have sampled ocean water and air for CO2 gas. But, sampling from moving vessels e.g., the many voyages of Charles Keeling and many others, or sampling in wind or ocean currents, or wave-disturbed surfaces, causes changes in CO2 partial pressure (and other variables) resulting in measurement errors. The rapidly reversible CO2 chemical hydration reaction occurs in all layers of the ocean and the CO2 physical phase state reaction occurs at all layers of the ocean and in the troposphere.
- Agitation of air or water increases their temperature as well as increases the surface area of the interface and this changes the net flux rate of the gas. The net flux of a gas through a surface interface is a function of the surface area of the gas/liquid interface, and a function of the thickness of that interface layer, and a function of the gradient of the gas in the interface layer, and a function of the Graham’s Law diffusion constant for the solute gas. Net flux is the vector directional residual difference between the molar mass of all solute gas emissions minus the molar mass of all solute gas absorptions passing through a known amount of surface area and known surface thickness in a known time period; for example gigatons of CO2 gas emitted into air per square mile of ocean surface per year. Net flux is directional (either absorption or emission.) Net flux is defined by Fick’s 1st Law. The CO2 concentration measured at NOAA Mauna Loa is a generally accepted defacto standard for the average net global CO2 concentration. The slope of that Mauna Loa concentration record with respect to time (often called the Keeling Curve) is the slope of the net flux of CO2 with respect of time, which is dominantly (~ 71%) the record of Fick’s 1st law for net flux CO2 gas and ocean. The Mauna Loa lab (and most NOAA and similar labs) measures by molar mass the net residual difference of CO2 gas emitted from all sources of CO2 gas natural and human minus all CO2 gas sinks natural and human; these labs do not and could not measure global human CO2 emissions, nor net human CO2 emissions nor net human CO2 flux.
The much higher density of water compared to the density of air causes much higher CO2 gas concentration in ocean water matrix compared to CO2 gas concentration in air. This occurs at all depths of ocean, regardless of mixing. Geology texts calculate the ratio of CO2 gas in ocean versus CO2 gas in air at about 50:1, which is a very broad average. The ratio varies with temperature and altitude (air pressure/density) and ocean depth (water pressure/density). Density of water increases rapidly with depth while density of air decreases with altitude but less rapidly. There are systemic processes as well as chaotic processes of mixing in both atmosphere and ocean. There is layering within both atmosphere and ocean and different physical and chemical reactions occur in the different layers. The less-well mixed deep ocean layers contain much higher molar concentration of CO2 gas than the well-mixed medium ocean layer. The more well mixed surface ocean layer (which is usually but not always warmer than the layers below) contains lower concentration than the ocean layers beneath it. Theoretically at the ideal limit, at the air/ocean surface interface, in the ocean thin film layer of a few molecules thickness, in calm flat seas with no water current and no wind, the Henry Law constant for a given ocean surface temperature determines the molar concentration of CO2 in the water surface divided by the molar concentration of the CO2 in the air thin layer above the surface; practically, this ideal theoretical condition does not exist except in transition.
The hypothetical equilibrium condition for Henry’s Law means that the ratio of the number of gas molecules of a specific gas being absorbed by a liquid surface versus the number of molecules of the same gas being emitted from that liquid surface is a constant for the given temperature of the surface. Unlike total pressure of mixed gases where at equilibrium the amount of total gas emitted equals amount of total gas absorbed, instead, Henry’s Law applies to partial pressure of a single specific gas, wherein, the ratio absorption vs emission of the single gas is the Henry’s Law partition ratio, or coefficient. Absorption and emission are occurring simultaneously, but not at the same rate. The absorption rate of a specific gas like CO2 into water surface can be increasing while simultaneously the rate of emission of CO2 from the nearby water surface can also be increasing; the conditions causing these two opposing perturbations to the Henry’s Law partition ratio for CO2 gas and water are different in the gas matrix and the liquid matrix.
When CO2 is added to air by burning fossil fuels, other sources of CO2 are naturally reduced and/or sinks are naturally increased so that the Henry’s Law ratio is maintained for the surface local temperature. It is a dynamic equilibrium and the control knob for the gas concentration, if you will, is the Henry’s Law ratio at the local surface temperature. CO2 is not accumulating in the atmosphere as hundreds of scientists and millions of dollars spent on annual carbon budgets attempt to persuade. CO2 has been increasing slowly in recent years because sea surface temperatures (SST) have been increasing in recent years. If and when SSTs begin decreasing, the CO2 concentration in air will also begin declining, though this decline is slow due to the enormous (orders of magnitude) higher heat capacity of ocean compared to atmosphere. For now, slowly warming SST and rising CO2 are both good for life.
Warming and cooling of ocean are very slow processes requiring centuries or millennia due to the enormous heat capacity of the ocean. The causes of warming and cooling are beyond the scope of this email except to point out firmly that they are definitely not caused by human-produced CO2;this statement does not require refutation or denial of the so-called “greenhouse gas” theory, radiative emission theory, carbon budgeting, feedbacks, etc.
The Henry’s Law coefficient is usually defined as kH = ca/pg
Where:
- kH refers to standard conditions, T = temperature = 298.15 K = 25 degrees C, and total pressure is 1 atm.
- ca is the concentration of the unreacted solute gas in theliquid phase
- pg is the partial pressure of the same solute gas in the gas phase incontinuouscontact with the surface of the liquid phase.
This version of Henry’s Law above requires calculations and measurements in volume for pg.
One of the common problems encountered with Henry’s Law is its dependence on temperature. kH is a constant (or coefficient, or ratio) that varies with temperature. This is not a simple matter. For example, one of the teachers for chromatography classes for the American Chemical Society, who was one of the founding fathers of gas chromatography, published errors of Henry’s Law constants derived for temperature ranges; he was not alone. No doubt this author’s own writings on his personal web blog have errors too. Derivation of Henry’s law constants as a function of temperature is based on solution of the Clapeyron or Van Hoff equations formulated for water-gas equilibrium. Great care is required with the units.
dln kH/dT = ∆Hdis/RgT2
Where:
- ∆Hdis is the enthalpy (or heat) of dissolution of the gas solute in water
- Rg is the gas constant
- T is temperature in Kelvin.
Henry’s Law constant for CO2 gas and water solution. (as given in reference 5 below)
kH(T) = k°H exp(d(ln(kH))/d(1/T) ((1/T) – 1/(298.15 K)))
k°H = Henry’s law constant for solubility in water at 298.15 K (mol/(kg*bar))
d(ln(kH))/d(1/T) = Temperature dependence constant (K)
For our purposes studying CO2, atmospheric gases and Henry’s Law, a different derivation of Henry’s Law is much more convenient. Sander provides this derivation also: the Henry’s Law coefficient is derived in its dimensionless (or unitless) form as the quotient (or ratio) of the molar concentration of the unreacted solute gas dissolved in the liquid phase matrix divided by the molar concentration of the same solute gas in the gas phase matrix. It is dimensionless since the units drop out when dividing molar mass by molar mass. But, the temperature derivation is still difficult. Today fortunately we look up Henry’s coefficients in reference books or by online software and then carefully verify the units. There is a different kH for each gas and liquid combination and each temperature.
Henry’s Law expert Rolf Sander provides further expansion of various derivations in section 4.2 of the IUPAC Recommendations 2021 paper with other detail on the other forms of Henry’s law for various purposes here: https://www.degruyter.com/document/doi/10.1515/pac-2020-0302/html and references (4) and (5) below.
These two equations for Henry’s law, the partial pressure version above and the dimensionless version below, are related through the ideal gas law, where kH = kH x RT.
The dimensionless version:
kH = ca/cg
Where:
- kH = the Henry’sLaw coefficient
- ca = concentration of the gas in moles in the aqueous phase
- and cg is concentration of the same gas in the gas phase above the liquid/gas surface.
Generally, the dimensionless version is most convenient because the defacto NOAA-Scripps Mauna Loa “gold standard” CO2 data has been diligently measured and reported for many decades as micromoles of CO2 per mole of dry air. Micromoles of CO2 per mole of dry air is identical to ppm, parts per million by mass. This is a mass measurement, not a volume measurement, which is far more practical, precise and accurate for measuring trends in atmospheric gases since air always contains the gas water vapor, the quantity of water vapor in air is highly variable which causes the sample volume to be highly variable. Water vapor concentration in air is normally 10 to 100 times higher than the trace gases like CO2, methane (CH4), etc. Also, water vapor and CO2 have overlapping wavelengths of infrared light used in measurement instrumentation. These are reasons NOAA-Scripps dries their air samples (by freezing) and measures by mass instead of volume, i.e., micromoles of CO2 per moles of dry air which equals ppm.
Important note: ppm is not the same as ppmv. This is a common and large source of error in measuring net CO2 trends compared to the minor annual change in net global CO2 concentration.
Henry law coefficients are available online, having been measured thousands of times for thousands of combinations temperatures, liquid solvents and gas solutes. The concentration of CO2 in water is easily calculated from the gas concentration and the Henry’s coefficient, but the CO2 and H2CO3 (carbonic acid) concentrations are difficult or impossible to measure in water with acceptable accuracy and precision because sampling procedures affect the hydration reaction.
The gas-water surface interface can be the surface of a bubble at 3000 meters depth in ocean, or the surface of a raindrop in a cloud at 3000 meters altitude, lung tissue, leaf tissue, or dominantly the surface of the ocean.
In a mixture of gases, the flux of one gas back and forth across the surface does not affect the flux of a different gas until concentrations are very high. This was demonstrated by Adolph Fick, a physiologist, who studied air gas fluxes in lung tissues, a contemporary of William Henry, Thomas Graham, and John Dalton.
In nearly pure water like raindrops, the aqueous CO2 gas concentration is high and the H2CO3 (carbonic acid) concentration is high relative to the HCO3– (bicarbonate) concentration. Thus raindrops are measured slightly acid. Carbonic acid is a weak acid. But those raindrops also absorb other gases of nitrogen and sulfur (for example near an urban area or a refinery) which create strong acids in water. In contrast, in seawater HCO3– is the dominant species because there are very many more ionic species (sodium, calcium, etc.) dissolved in seawater which easily pull the first hydrogen ion away from the H2CO3. The concentration of calcium ion by itself is about 4 times as abundant in seawater surface as the combined concentrations of unreacted CO2 gas, bicarbonate ion, carbonate ion, and carbonic acid. The first dissociation constant for pulling away the first hydrogen atom from H2CO2 leaving HCO3– is very small and the reaction is nearly instant. So, dependent on the seawater conditions, HCO3– can be both a reaction product from the CO2 gas hydration reaction and also a reaction product from the dissociation reaction of H2CO3.


Graphics above from Andrew G. Dickson, Scripps Institution of Oceanography, UC San Diego, INTRODUCTION TO CO2 CHEMISTRY IN SEA WATER.(6)
There are many simultaneous phenomena occurring at these air/liquid surfaces. I have not touched on most of them. The most important quantitatively is the physical phase state reaction, wherein the change in partial pressure of the solute gas within the gas matrix causes a proportional change in the solubility of that unreacted solute gas in the liquid phase. When humans or any other source add CO2 to air, then the solubility of CO2 gas in water increases proportionally to absorb that CO2 gas. Double the partial pressure of CO2, then solubility of CO2 in water doubles. And vice versa.
The Henry’s Law ratio is a variable of surface temperature, not a variable of the amount of gas present, and not a variable of the source of the gas; it applies to all gas solutes in low concentrations with respect to the liquid solvents with which they are in continuous contact. The ratio does not apply to any portion of the gas solute which has reacted with the liquid solvent itself or has reacted with any component dissolved in the liquid solvent. Residence time, or half-life, or residual fraction, of the CO2 in air are not variables in the Henry Law phase-state equilibrium and play no role in net global CO2 concentration.
Next in importance is to understand that the CO2 hydration reaction (CO2 + H20 <-> H2CO3) is nearly instantly reversible, for example by a very minor increase in water temperature, or agitation of the water, or change in other variables. Then the first dissociation constant K1, which is dissociation on one hydrogen away from H2CO3, this K1 is small, yielding HCO32-. The subsequent K2 dissociation reaction to CO32- is relatively larger. Both reactions are nearly instantly reversible. But the hydration reaction CO2 + H20 <-> H2CO3 is so fast it is unmeasurable. Sampling changes the measurement. Unfortunately many textbooks merge unreacted aqueous CO2 gas and H2CO2 into a single hypothetical entity, which has apparently led to misunderstanding.
There is a highly abundant (>90%) reservoir of these readily available carbonate ions in seawater surface thin layer and well mixed layer which are not unreacted aqueous CO2 gas but which are very rapidly convertible to unreacted aqueous CO2 gas when the unreacted aqueous CO2 gas in seawater is depleted. And vice versa. We have shown this in Bromley & Tamarkin (2022). Depletion of CO2 in air as well as CO2 in water occurs by many different processes, and addition of CO2 to air and water occurs by many different processes. For example, outgassing of CO2 from water to air occurs to rebalance to Henry’s Law coefficient when CO2 concentration in air is being depleted by photosynthesis of land plants or by surface winds; conversely when aquatic plants like plankton bloom in ocean and absorb aqueous CO2 gas for their photosynthesis, then CO2 gas will be absorbed into water from air at higher ratio than is being emitted from water to air until the Henry’s Law coefficient is achieved for the water surface temperature. These opposing processes can and do occur simultaneously, which is probably a reason climatologist rarely if ever attempt to model Henry’s Law.
To summarize so far, when the approximately 1% of aqueous CO2 gas is either increased or decreased by any amount, due to any source or sink, this perturbation causes a recovery (following le Chatelier’s Principle) to rapidly return to that 1% by the using ocean’s chemistry systems, and there is an abundant reservoir of ionic carbonates in ocean water to achieve that recovery. In other words, the amount of CO2 emitted by humans replaces naturally emitted CO2 in the phase-state equilibrium, rather than accumulating in the atmosphere in addition to naturally occurring CO2. There is no CO2 accumulation in atmosphere. The ~1% can be perturbed in both directions by changes in temperature, salinity, pH, alkalinity, and by changes in partial pressure of the CO2 in the water or in the air. Adsorption and emission of CO2 are simultaneous and continuous in both directions at normal earth temperatures since CO2 molecules are continuously colliding with the surface and being emitted from the surface. The rate of absorption vs emission is the Henry’s Law coefficient for the local water temperature.
It is very important to mention that CO2 gas molecules and the three forms of carbonate ions (H2CO3, HCO32-, CO32-) do not need to migrate in water more than molecular distances to react with each other. Scientific publications which are adopted into the anthropogenic global warming (AGW) orthodoxy and climate models, for example work by (1) Bert Bolin (1960) first administrator of UN IPCC, and (2) Broecker and Peng (1974), and others, considered the thickness of the water thin layer and limited their calculus of aqueous CO2 migration to thickness of the water matrix. They define the chemical pathways that convert CO2 to HCO3– and vice versa as “chemical enhancement.” They calculate based on the thickness of the thin layer and migration time of the carbon species in the ocean matrix, however they ignore an equally critical variable which is the surface area of the gas – liquid interface, omitting more than 100 million square miles of air-water surface interface. This appears to be a major mistake in the AGW orthodoxy and modeling. Keep in mind that in these years of early papers seminal to IPCC orthodoxy, CO2 emissions were said to cause global cooling; I speculate they were focused on their modeling methods rather than empirical observations.
For example, “The rate limiting step for removal of anthropogenic CO2 from the air is vertical mixing within the sea rather than transfer across the air-sea interface.”(2) This statement conflicts with Fick’s 1st Law of net flux of gases.
Why did Bolin and Broecker & Pang (for example) consider only thickness of the ocean thin layer and not the huge square surface area of ocean? It is a puzzle. The 3 carbonate ionic species are distributed throughout the area of the thin layer as well as the well mixed layer beneath it and they surround the uncharged aqueous CO2 gas molecules in the thin layer and well mixed layer in a ratio of over 9:1. If the uncharged aqueous CO2 gas molecule is removed from the liquid matrix by either outgassing or by absorption into aquatic plants, then the carbonate reactions reverse and produce more uncharged aqueous CO2 gas molecules. And vice versa. And when the carbonate ion CO32- reacts with for example a very abundant ion such as calcium Ca2+ and solidifies, then the carbonate ion in water is thus reduced one for one. CO32- +Ca2+ = CaCO3 . Then as a consequence of this reaction (which occurs in warmer water near the surface, and reverses in colder deeper, higher pressure water), the prior carbonate and the hydration reactions are forced to re-equilibrate by absorbing more CO2 from air. Thus, there is a perpetual CO2 gas sink rate absorbing CO2 from air even while warming SST is slowly increasing outgassing CO2. These simultaneously opposed dynamic processes are mediated by Henry’s Law and the carbonate reactions.
Attached is a pdf (reference 3) which documents one of several gas chromatographic (GC) methods to quantify Henry’s Law coefficients. To use this method for CO2, since CO2 does not burn in the flame ionization detector (FID) flame, instead the effluent from GC column is passed first over a rubidium catalyst which stoichiometrically converts the CO2 to CH4, and then the effluent is directed onward to the FID where the CH4 is ionized and quantified. Alternatively, the FID can be replaced by a mass spectrometer and no catalyst is needed. I am pointing out that there is no mystery in Henry’s Law, there are many of these methods well documented by theory and experiment, but AGW proponents either ignore it or fail to understand it or both.
On the Exchange of Carbon Dioxide Between the Atmosphere and the Sea (PDF)
Gas Exchange Between Air and Sea (PDF)